Bryan Dyne
The Nobel Prize in Physics for 2017 has been awarded to Kip Thorne, Rainer Weiss and Barry Barish for their pioneering work to directly observe gravitational waves. As noted by the Nobel committee, this was a decisive advance in scientific cognition and technical mastery and has been celebrated around the world.
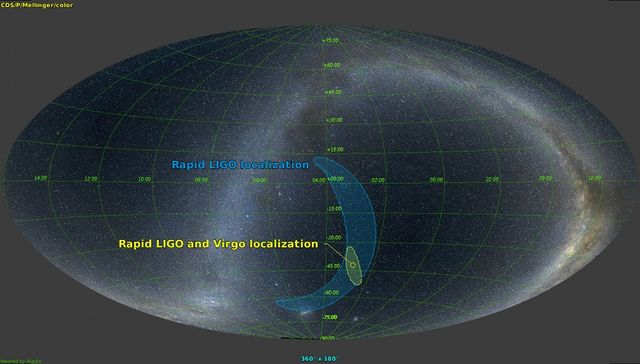
The work spanned the course of five decades and culminated on September 14, 2015 when the twin Advanced Laser Interferometer Gravitational-wave Observatory (LIGO) detectors recorded the first unambiguous signature of a gravity wave—one which also matched theoretical predictions of what a merger of two black holes into a larger one would show. Gravitational waves were first predicted by Einstein in 1916 and have been pursued for the past century as one of the many proofs of the general theory of relativity, and as a tool to explore a new facet of the universe.
Those honored in the prize ceremony reflect some of the variety of backgrounds of the nearly 1200 researchers and engineers that work on LIGO. Thorne was born in Utah in 1940 to a Mormon family whose ancestors traveled west by foot and horse during the era during and after the American Civil War. He studied general relativity at Princeton and joined the faculty of the California Institute of Technology in 1967 where he began to work on the mathematics needed to detect gravitational waves. Weiss and his family were forced to flee Nazi Germany and then Europe in 1938, when the future physicist was six years old, so that his father could avoid persucution and possibly death at the hands of the Nazis for being Jewish and for being a member of the German Communist Party. His academic career, from his bachelor's through his professorship, centered around the Massechusetts Institute of Technology, where he started work in the 1970s to develop the special instruments needed to test general relativity. Barish, now 81, was born in Nebraska to Jewish immigrants from Belarus. His education and professional life have been at CalTech, where he is currently a professor emeritus and the principal investigator and director of the LIGO collaboration.
Two others, both deceased and thus ineligible for the Nobel, deserve mention for their critical contributions to LIGO: Ronald Drever and Vladimir Braginsky. Drever was born in 1931 in Bishopton, Renfrewshire, Scotland and received both his bachelor's and doctorate at the University of Glasgow. He was part of the original team in the 1970s that worked on the mathematics to analyze and detect gravitational waves. Braginsky was born in Moscow in 1931 and received his Ph.D. from the Moscow State University in 1967. He had been working on his own method to detect gravitational waves when he was visited by Kip Thorne in 1968 and the two worked closely until Braginsky's death in 2016.
Two others, both deceased and thus ineligible for the Nobel, deserve mention for their critical contributions to LIGO: Ronald Drever and Vladimir Braginsky. Drever was born in 1931 in Bishopton, Renfrewshire, Scotland and received both his bachelor's and doctorate at the University of Glasgow. He was part of the original team in the 1970s that worked on the mathematics to analyze and detect gravitational waves. Braginsky was born in Moscow in 1931 and received his Ph.D. from the Moscow State University in 1967. He had been working on his own method to detect gravitational waves when he was visited by Kip Thorne in 1968 and the two worked closely until Braginsky's death in 2016.
From a technical standpoint alone, the achievement of the LIGO collaboration is deserving of the prize. Starting in the early 1990s, the detectors were developed with the understanding that new technologies in lasers, vacuums, remote sensing, mirror polishing and seismology would all be needed to achieve the necessary sensitivity. The hundreds of engineers on this project played critical roles in trailblazing new fields to complete the first iteration of this detector, and to further upgrade it to sensitivities which now permit “routine” detection.
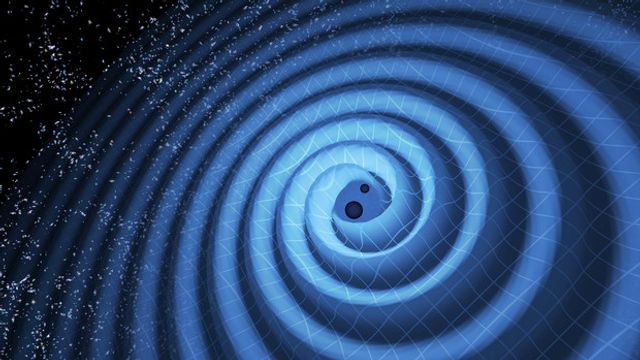
Researchers on the project also had to develop new areas of mathematics to properly understand the signals that were recorded and to extract an actual gravitational wave signature from the myriad false signals that appear. In effect, they had to measure the distance between Earth and the nearest star, Proxima Centauri, to the accuracy of the width of a human hair, while at the same time accounting for the subtle shifts in Earth's orbit caused by every planet, moon, asteroid and comet in the Solar System.
The theoretical achievement, however, is even more profound. The vast majority of what humanity has learned about what exists beyond Earth has been through the study of light and its different wavelengths and orientations. On occasion, we have learned about high energy processes when Earth is struck by a particularly intense cosmic ray, a particle produced vary far away and by some colossally powerful event such as a gamma ray burst. Once, in 1987, 20 neutrinos were detected from a star as it collapsed into a supernova, confirming parts of our understanding of the last moments of the life of stars much more massive than our own Sun.
Now, however, astronomers have a growing data set of events only observable through their gravitational interactions, through the warping of space and time that is caused as objects accelerate. While this field is still basically brand new, it has already yielded an impressive amount of scientific results.
To date, there have been four concrete detections of gravitational waves and a handful more that are less statistically certain. One of the initial findings is that all the events detected so far are from the merger of black holes in the range of seven to 36 times the mass of the Sun. While the existence of these objects had been considered a possibility, they had never been previously observed and no one was sure of how common they were or how often they collide. The origin of this population remains uncertain—some think many may arise from the very first population of stars. Thanks to LIGO and its sister detector Virgo, cosmologists now have new windows into the formation and evolution of rare ultra-heavy stars, addressing some long-standing questions.
There are also tantalizing clues, which a larger sample of detections will pin down, as to whether this first generation of stars formed preferentially in dense clusters or was more evenly distributed. The detected black hole collisions also give clues about how the supermassive black holes formed that are found at the heart of virtually every galaxy.
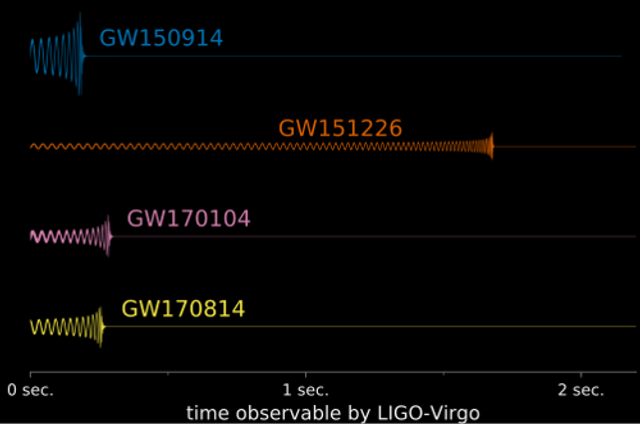
The most recent detection, recorded seven and a half weeks ago on August 14, has revealed even more. This was the first time that the two LIGO detectors, in Hanford, Washington and Livingston, Louisiana, were able to work in conjunction with the newly upgraded Virgo Observatory located near Pisa, Italy. By utilizing the detection recorded by all three instruments, the astronomers on each project were able to shrink the volume of sky from which the gravitational waves originated by a factor of 20. This enabled 25 facilities from around the world to perform follow-up visual observations, but no bright event was identified in the calculated region, further confirmation that what is producing the gravitational wave is a black hole merger.
The three-detector observation has also allowed researchers to determine the internal oscillation structure (the polarization) of the gravitational wave. This was a critical test of general relativity, which predicts a certain description of gravitational waves. Other theories of gravity, which modify, expand or sometimes replace general relativity, have their own predictions. The current analysis shows that even in such extreme cases, Einstein's theory is correct.
One of the more exciting future prospects about gravitational wave astronomy is the ability to directly gather information further back in time than is possible with light. As anyone who has ever stood in the shadow of a tree on a sunny day understands, branches, leaves and a variety of types of matter can block light. This is replicated on a cosmic scale when attempting to use light to see the first moments of the known universe. There is a "shadow" known as the cosmic microwave background that prevents us from seeing the first 380,000 years of the known universe's existence.
Gravitational waves do not have this problem. As far as we know, the only object that might reflect, refract, diffract or absorb a gravitational wave is a black hole, and they are few enough in number compared to the vastness of space that gravitational waves travel essentially unimpeded from where they were created to Earth. This allows us to get first-hand knowledge from events that we literally cannot see.
While a great deal has been learned in the past two years, a great many more questions remain. For example, there has yet to be a detected gravitational wave signal from a collision involving neutron stars or white dwarfs, both extremely dense objects whose insides are much more complicated than that of a black hole. And LIGO and Virgo themselves only observe one part of the gravitational wave spectrum.
A different sort of detection method, known as pulsar timing, observes different frequencies of gravitational waves than those observed by LIGO and Virgo and is expected to find gravitational waves from supermassive black holes during galactic mergers. As more and more instruments to detect gravitational waves come online, the more humanity learns about some of the most esoteric events in the cosmos, including its origins.
No comments:
Post a Comment